Future Battery Materials And Technology Innovations
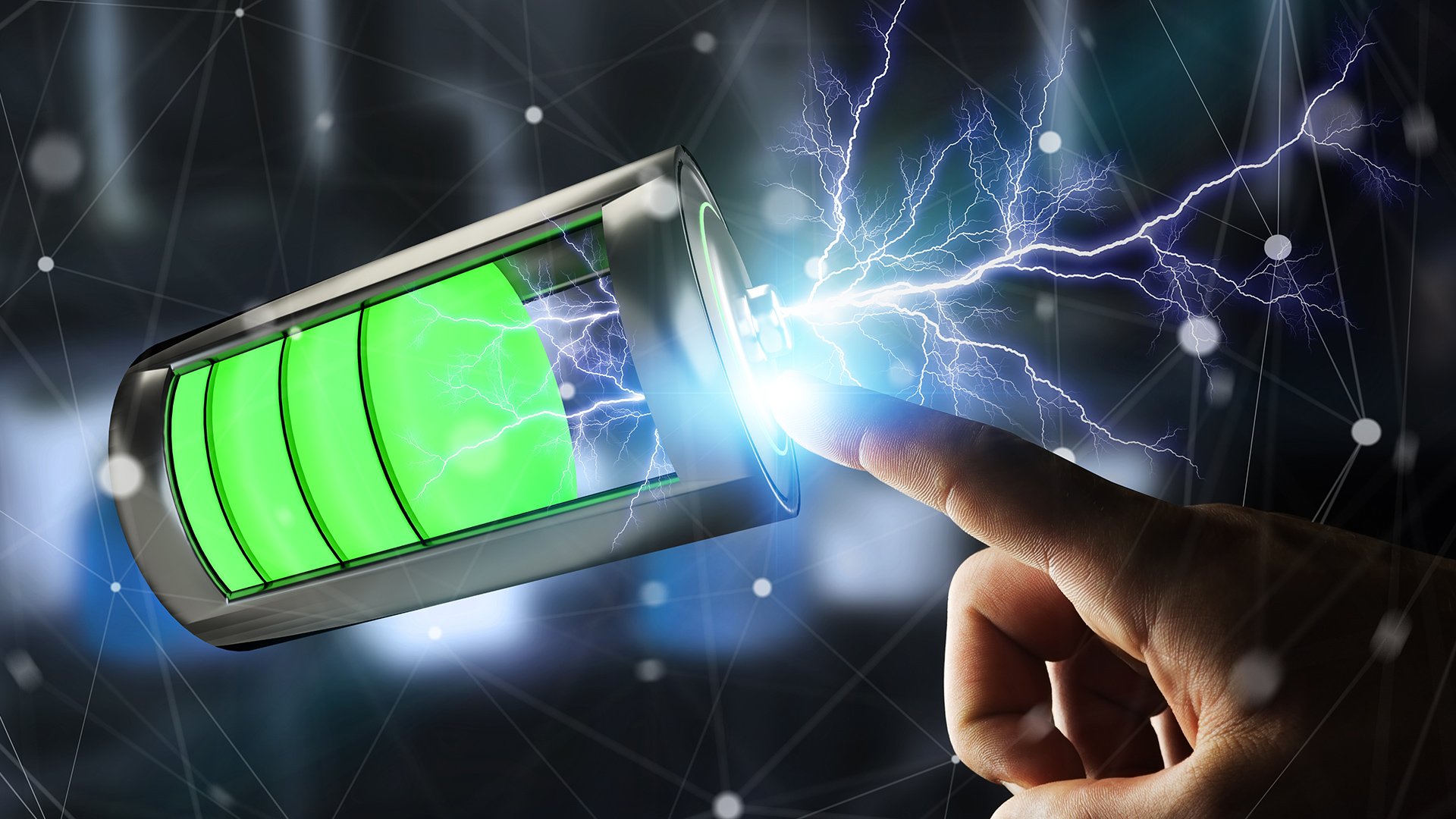
From portable tools to computers and mobile phones, and from uninterruptible power sources to satellites, batteries have always been an important design aspect. For years, scientists have been working on ways to boost the energy density of batteries (the amount of energy in a given size and weight). The demand for higher energy densities arose as the number of handheld devices increased, ranging from industrial measuring equipment to mobile phones.
As the number of telecommunications satellites grew, battery weight became a consideration. Every technology improvement has tended to prioritize battery capabilities. While researchers worked to improve battery technology, electronics continued to evolve at a breakneck pace, necessitating ever-increasing amounts of energy and power.
The Current State of Batteries
Lithium metal, thionyl chloride (Li-SOCl2), and manganese oxide are used in today's state-of-the-art primary battery technology (Li-MnO2). They are appropriate for five to twenty-year applications, such as metering, electronic toll collecting, tracking, and the Internet of Things (IoT).
Lithium-ion batteries have been driven to reach higher and higher energy densities as the market for mobile phones, tablets, and laptop computers has grown. The amount of hours a battery can run is directly proportional to its energy density. Experts in the field have tweaked the technique to achieve higher densities, including changing chemistries and tweaking designs. They've even looked into the raw material supply chain, as cobalt as an addition to Li-ion designs is pricey and difficult to come by.
Power density, in addition to energy density, is a significant concern. The pace at which a battery may be drained (or charged) is measured by power density, whereas the total quantity of charge is measured by energy density. In comparison to a high-energy battery, which takes hours to discharge, a high-power battery can be depleted in just a few minutes. The trade-off between energy density and power density is inherent in battery design.
NEW GENERATION LITHIUM-ION
The flow of lithium ions from the positive to the negative electrode back and forth via the electrolyte provides energy storage and release in lithium-ion (Li-ion) batteries. The positive electrode serves as the initial lithium source, whereas the negative electrode serves as the lithium host in this technique. Li-ion batteries are a collection of chemistries that are the result of decades of careful selection and optimization of positive and negative active materials.
Li-ion battery technology currently has the highest energy density of all the state-of-the-art storage technologies. Furthermore, Li-ion batteries have a low self-discharge rate and a long lifetime and cycling performance, with thousands of charging and discharging cycles on average.
LITHIUM-SULFUR
Lithium ions are kept inactive materials in Li-ion batteries, which act as stable host structures during charge and discharge. There are no host structures in lithium-sulfur (Li-S) batteries. The lithium anode is consumed during discharging, and sulfur is converted into a variety of chemical compounds; when charging, the process is reversed.
The active components in a Li-S battery are extremely light: sulfur in the positive electrode and metallic lithium in the negative electrode. This is why its theoretical energy density is four times higher than that of Li-ion batteries. As a result, it's a suitable fit for the aerospace and aviation sectors.
SOLID-STATE
Solid-state batteries constitute a technological paradigm leap. Ions migrate from one electrode to another across the liquid electrolyte in modern Li-ion batteries (also called ionic conductivity). The liquid electrolyte is replaced by a solid component in all-solid-state batteries, but lithium ions can still travel through it.
The first major benefit is a significant increase in cell and battery safety: solid electrolytes, unlike liquid electrolytes, are non-flammable when heated. Second, it allows for the use of cutting-edge, high-voltage, high-capacity materials, resulting in denser, lighter batteries with longer shelf lives due to reduced self-discharge.
Why Shift to Solid-State Batteries?
For a variety of reasons, the industry is currently migrating to solid-state batteries. The first is that, even when fine-tuning the design to acquire higher density, ordinary lithium batteries with a liquid electrolyte have reached the theoretical limits of the electrode combinations being employed. However, with the popularity of electric vehicles (EVs) growing, there is a great need for ever-increasing energy densities, with each rise directly linked to better vehicle range and battery life in general.
The need for significantly greater capacity electrodes, such as solid lithium metal, means Watt-hour per kilogram gains of 50 to 100 percent are possible. Additionally, replacing the volatile and flammable liquid electrolyte with a stable and solid substance that will not exhibit the thermal runaway problem experienced in the past—for example, solid lithium-ion is far safer chemistry.
Solid-state batteries face technological obstacles in addition to addressing issues of price and scale. Solid-state batteries are safer, although dendrites, the root-like build-up on lithium metal in the anodes that occurs as the battery charges and discharges, still exist. The amount of solid electrolyte capacity and hence the stored charge is reduced when dendrites form.
Promising battery technology
As previously said, EV adoption is acting as a driver for battery sector development and vice versa. A Tesla Model S 85-kWh Lithium-ion battery, for example, weighs around 1,200 pounds and has 7,104 cells. It has a great range of up to 265 miles, however, charging it at a regular 220V source can take up to 3-4 hours.
The first million-mile battery' was announced by a Chinese battery manufacturer that supplies most of the major automakers (including Tesla). Over a 16-year lifespan, Contemporary Amperex Technology (CATL) claims that its new battery can power a vehicle for more than a million miles (1.2 million, to be exact – or 1.9 million kilometers).
Echion Technologies, an English start-up, claims to have created an anode for high-capacity Lithium batteries that cuts recharging periods in half. The anode is known as a mixed niobium oxide anode because it functions as a negative pole during use and as a positive pole during charging. To improve recharging, the mixed niobium oxide anode can be substituted for any other anode style. It may be used with traditional cathodes and electrolyte materials, making it widely applicable. Mixed niobium oxide anodes make the audacious claim that they can recharge high-capacity Lithium batteries in as little as six minutes.
The Porsche Taycan, Porsche's effort at a high-performance electric vehicle, has a 93.4kWh battery that generates 800V rather than the typical 400V seen in most other electric vehicles. The Taycan's battery is made up of 33 battery modules, each with 12 cells, for a total of 396 lithium-ion cells with a storage capacity of 235.8 Wh per cell. Because current limits battery charging speed, the higher voltage these cells provide means lighter battery systems and faster charging.
Conclusion: The Future of Energy Storage
The eventual economic ramifications of electric automobiles, flying vehicles, more efficient energy storage networks, and silviculture are difficult to foresee or even envision at this stage; we can't help but make wild assumptions and envisage future solutions for tomorrow. The way we design, manufacture, and use batteries in the future appears to be one of the major catalysts for the eventual transformation of our relationship with energy; this could actually bring humanity one step closer to becoming an eco-friendly species, rather than carving and burning the planet to power itself.
Small cells have already demonstrated the increased capabilities required of solid-state batteries, so it's only a matter of time before larger batteries' production processes catch up. Several businesses have predicted that some of these batteries will be available as early as next year, while others will be available by 2025. Technological advancements, like liquid electrolyte Li-ion batteries, will push us forward once manufacturing catches up. This means that materials and design approaches will likely be tweaked in the next years to advance battery capacity.